Can Corals Resist Bleaching?
This month, corals that can resist bleaching, signs that the human immune system went up a gear about 8000 years ago, documenting plant cells with an ambitious initiative to generate an atlas all the cell types in all types of plants, new insights into the science of the hug hormone oxytocin, and how deleterious genes hold up the evolution of healthy genes too...
In this episode
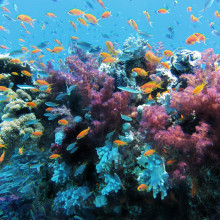
00:39 - Corals Resistant to Bleaching
Corals Resistant to Bleaching
Brendan Cornwell, Hopkins Marine Station
One of the starkest reminders that the world is warming is the mass “bleaching” of corals. This happens because, when the water temperature climbs, the corals part company with symbiotic algae that live inside their cells and provide oxygen and remove waste. Their departure kills the coral. Why higher temperatures should cause these two partners to divorce isn’t known, but scientists have discovered that some corals are more immune to this effect than others. Those with fewer algae seem to be more tolerant, but the tradeoff might be slower growth and therefore less resilience to other threats. Speaking with Chris Smith, Brendan Cornwell has been studying this both in the lab and on reefs for real…
Brendan - We actually have to go and get in the water. So we want to know both what the temperature conditions are, so we deploy data loggers to each of these reefs to figure that out. So they basically, we zip tie them to the rock and then they sit there for the entire year, or a few months, constantly recording the temperature, and then we go get them. But then the other thing we want to know is how the corals react to a really standardised set of conditions. So we actually have to heat them up ourselves and bleach them, and ask how much they bleach when we give them a specific amount of heat stress, in order to identify more or less bleaching resistant populations.
Chris - So you've got real world data of what's going on, where you see the bleaching. But you've also got samples that you can subject to conditions that enable you to work out just how much they'll take before they bleach. So you can pinpoint where it's likely to be worse, where it's likely to be better.
Brendan - Exactly. And the sort of nice thing about it is that these are colonies of polyps, right? So the coral itself is made up of lots and lots of different polyps. They're all clonal. So they're all genetically identical and they're all making up the colony itself. And so when you take a little piece of the colony, you don't actually kill the entire thing. You just take what we call a nubbin, and that nubbin is what we're actually doing the experiment on. And so what that allows us to do is both figure out what that colony can and can't stand in terms of heat stress, but also it lets us go back to that colony over and over again, and ask if that changes.
Chris - Right. So if one looks at the corals that are coming out of these experiments as clearly more resilient, compared with corals that are more vulnerable to bleaching at temperature escalations. When they do this, is there any difference in terms of the performance of the corals, when they're growing, when they're not trying to bleach?
Brendan - That is one of the questions that we've started to ask ourselves, is essentially what do you gain by being bleaching resistant and why hasn't bleaching resistance just spread throughout the population, if it's so favourable when conditions warm? Part of what we found in this study, is that we think we see a trade off between how fast the coral can grow and how readily it bleaches. We think that has to do with the overall abundance of symbiont in the coral tissue itself. So how much of those algae, how many are actually in the tissue? We think that matters for both how severe the bleaching is, but also for how quickly they grow. So we think when they have more algae, what we're seeing is that their growth rate is slightly higher than if they have fewer algae, which logically makes some sense.
Chris - Indeed. So what one would anticipate then is that the ones that have fewer algae are going to grow more slowly, but they're going to be a bit more resistant.
Brendan - Exactly, yeah. They'll lose overall, as a proportion of the initial population that they were harbouring, they'll lose fewer of those.
Chris - Now, if the motivation for doing this is to study corals that are more bleaching resistant, and then perhaps even use those to repopulate decimated reefs elsewhere, is there not a danger then that we could be selecting subpopulations of coral that actually are going to be much slower growers? So our recovery times might be quite a lot longer?
Brendan - Yeah. I think that is definitely a possibility and it might not be something that we can necessarily overcome, but might just have to be something that we kind of know is coming. So yeah, it could be that we select for these bleaching resistant colonies that are more slow growing, which will mean that they won't be able to deal with the other stress that we might lay on them. It could be that they overall are just slower, moving into the future, because they have to adopt that strategy in order to not face such severe bleaching when temperatures warm up.
Chris - Are you seeing a sort of overgrowth of the resistant forms, sort of moving into the space vacated by what would've been their faster growing, but more vulnerable cousins, which have now obviously been killed off in some places by bleaching events?
Brendan - We are not. So we have not been at this particular location for long enough, I think to have seen that. But there are now indications in the literature that something like that might be happening. Essentially that we're imposing such strong selection on these populations, that we are seeing an evolutionary response. It's really difficult to know whether or not that is strictly due to inheriting that response from your parents, or if it's due to some sort of acclimation. But there are indications now from studies on the great barrier reef, that there is some sort of shift in terms of the overall bleaching resistance and resilience of the populations, which, that's definitely one reason why that might be the case.
Chris - And are the resistant forms sort of represented across coral populations, or are we looking at a future where we have a much more restricted pool of species, which are the resistant ones, but which in turn might not actually therefore support such a diverse reef, and all of the other attendant species that would normally make it their home?
Brendan - That's hard to know, but to me, it seems reasonable to think that some of the more bleaching prone species will likely be the first to be lost. So I do expect that the composition of these reefs, in terms of species that you can find there, will shift as temperatures warm. I think that's probably inevitable.
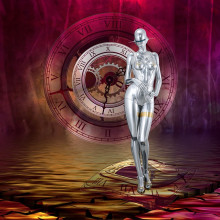
06:45 - Human Immunity Evolution
Human Immunity Evolution
Mihai Netea, Radboud University Nijmegen
When we come into contact with an infectious disease, our immune system is usually able to detect and destroy it. Indeed our bodies can tackle a wide variety of microbes. But it wasn’t always this way - we think our immune systems went up a gear about 8000 years ago. But how do we know this, and what gave human immunity this shot in the arm? Julia Ravey heard from Mihai Netea, from Radboud University, Nijmegen, who’s been using ancient DNA to find out...
Mihai - Our immune system is made to defend us against pathogens, and evolves depending on the interaction with these microorganisms. What is very important to realise, is the fact that along history, we changed a lot the infections with which we interact during our everyday life. 8,000 years ago we developed domestications of animals. And because we started to live very close to animals, the infectious burden very strongly increased, and the types of infections also changed, because now we took many more infections from the domesticated animals.
Julia - We have really great tools now for studying people's immune systems, but it's a bit harder to study people who lived 8,000 years ago. How did you investigate how our immune response evolved?
Mihai - Well, indeed, it's very difficult to study the immune system because we do not know those people who lived so many years ago, and we don't have their cells to study, and so on. However, one thing that we can study is how the genetic code, how our DNA changed during this period. Because now we know exactly what are the regions of the genomes, which are important for host defence. And we can see there, how those regions, important for host defence, have changed during the last couple of thousand years. And depending on how these changes took place, we can make predictions, whether our immune system works stronger or less strong or more tolerant against certain pathogens.
Julia - How did you find DNA from people who lived so long ago?
Mihai - Of course this is also not easy. And fortunately in Europe, we are lucky because of the type of climate that is here, which is not very, very hot. And especially for the bones that have been found, for example, in caves, which are in a natural refrigerator, let's put it this way. So we can isolate from small quantities of bone, especially from teeth, DNA, purify it and sequence it, and read precisely how does it differ from the DNA of the modern people?
Julia - The one perk of having bad weather is good preservation of DNA.
Mihai - Exactly.
Julia - By using our DNA as a sort of time machine, what did your research find?
Mihai - So what we have identified is the fact that our DNA and immune response changed significantly during the neolithic time period. So after the neolithic time period, when we came into contact with many more infections than previously, and that changes the way in which the immune system reacts. And in parallel with these changes that took place to help us in the fight against infections, it became clear that also the susceptibility that we have to certain immune mediated diseases that we have in modern times has changed as well. Just to give you an example, certain autoimmune diseases, such as multiple sclerosis or rheumatoid arthritis, which are very prevalent in the circulation. We would think that we are much more susceptible to those now, than thousands of years ago. Well, we discovered that that is not the case. In fact, the susceptibility to rheumatoid arthritis and multiple sclerosis decreased. That is not to say that nowadays less people have these diseases that they had previously, because of course thousands of years ago, people did not reach an age that they could develop these diseases. But in any case, our susceptibility to these diseases decreased. On the other hand, and this has to do probably with the type of diets that we had after domestication of animals and agriculture, we see an increase in susceptibility to inflammatory diseases of the gut. And this is very clear information that we obtain from these genetic studies.
Julia - It's really interesting how these results, from looking at our immune system responses thousands of years ago, can tell us information about potentially how we respond to disease causing bugs today. Do you think this work can be used in that way?
Mihai - Well, I do think that it makes us understand, what are the factors that are important for the susceptibility to infections, to inflammatory diseases, to other types of immune mediated diseases. And from drawing these conclusions, we can learn much better also nowadays, in what directions should we look for new types of therapies for the modern diseases. In the next period, we will investigate of course, how these differences in immune system change also between different populations around the world. This is something which is very important, because it tells us a lot about public health measures. How can we help people from various parts of the world? Where is the susceptibility higher or lower? How to predict what will happen in the future, because of the historical trends that are ongoing in the modern world. And hopefully this would help measures at the public health level to improve health in the populations. It's fascinating to have a mirror in the past, but at the same time, we try to help future generations with that, of course.
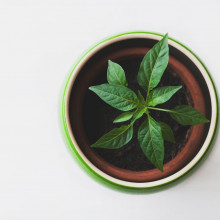
13:08 - Plant Cell Atlas Launch
Plant Cell Atlas Launch
Sue Rhee, Carnegie Institution for Science
If you want to know how something works, usually a machine, you open a workshop manual, which displays all the components and how they fit together. So it's logical that we should want something similar for living systems, isn't it? And biologists are busy building the same sort of thing for the human body, mapping out the chemical and genetic nuts and bolts of each individual cell type and how they work together. Surprisingly, though, that's currently not being done for the very thing that keeps the planet alive and probably holds the key to our - and the planet's - future health. I'm referring, of course, to plants; and, as she explains to Chris Smith, this is a shortcoming that Sue Rhee, from the Carnegie Institution for Science, wants to change...
Sue - Our vision is to create the plant cell atlas. We're hoping to achieve a community of plant biologists, data scientists and technology developers, who are interested in figuring out how plant cells work, so that ultimately we can maybe build or engineer plant cells.
Chris - Call me slightly naive, but I'm quite surprised that this sort of initiative doesn't exist already, because we have got similar atlases for say, human cells, haven't we? I know Sarah Teichmann at the Sanger has been helping to lead that project.
Sue - Yeah, the human cell atlas project really got started about five years ago. So it's not been that long since this concept of cell atlases has been around.
Chris - Is it that you're going to do things differently and do new things, or is it that there are people already doing a lot of this stuff, but they just need a framework to peg it to? So that you are going to deliver all of this in a very organised way, and therefore make the gaps a bit easier to see. So we know what we need to go after?
Sue - It's a little bit of both, but I would say by far, the majority of plant biologists are not looking at things at this level. And for those who are already doing it, I would say that they're doing it more or less on their own. So there isn't really a community supported standards or rubrics of how we should be defining cells, and states of cells in plants. I would say, we need to do both.
Chris - How's it actually going to work then? Talk us through what exactly the nuts and bolts of this are going to be. So someone coming from outside seeing this in five years time, what will they experience?
Sue - First of all, I hope that there will be a very easily accessible web portal, where anyone out there interested in plant biology could access all of this data. This portal, we hope, will present single cell information down from molecules, atomic level, all the way to organ and tissue levels.
Chris - Is this then that you will say, right, we're going to pick representative species from different groups of plants, and we're going to get representative cells from representative tissues in those things and then we're going to read every single gene that's in there, and how active it is for example. Is that kind of the level of resolution of what you're going for?
Sue - Yeah, exactly. I think that would be a really great place to be. But ultimately, you know, plants are quite diverse, you know, even just flowering plants, there are over 400,000 species. It would be really great to find these focal reference points, where we can get down to that level of resolution. But ultimately it would be great to be able to expand that, to represent the diversity of plants.
Chris - Presumably quite a lot of this data do exist for some plants already. And some of it you're going create de novo. So is part of the project going to be going and finding what's already out there and onboarding it, as it were. Bringing it within the portal, so that it's then in the right format, the right context, and you can see what still needs to be to done. And at the same time also showing people where the new avenues, the new vistas to explore are?
Sue - Yeah, that's exactly right. Yeah. There are already some data out there that we want to bring together, and identify what should be considered minimal requirements in describing the data.
Chris - A couple of things sort of spring to mind off the back of this. One, it's a huge undertaking. I mean, as you say, there are hundreds of thousands of plant species, and if we want to be comprehensive about it, this is a big undertaking. This is a long-term project, isn't it? So how are you actually going to do this? There must be some bottlenecks which are going to hold this up, which you are going to have to solve along the way. And how long is it going to take to do all of this?
Sue - Yeah, that's a really good question. I think there are mainly two bottlenecks that we need to overcome, and we need to overcome them pretty fast. The biggest bottleneck that I see, is that there are just very few plant scientists. In the US only about 10% of those working in the field of medicine are those that work in plants. We need to really increase the number of people working on plant biology. The second bottleneck that I see is that most of the genes in these plant genomes, or plant species, are still completely uncharacterised and unknown. So we need to find ways to try to find what these genes are doing in plants.
Chris - And the time scale?
Sue - It depends on several factors, but if we can excite new generations of scientists into plant biology, and if we can excite the general public and the politicians to funnel more funds into plant biology, then I think we can achieve it in a relatively reasonable time. But it all depends on what's available.
Chris - And speaking of what's available, who's actually putting up the funds? I mean, this is a stupendously big project, which is going to run for many years. So how much are you going to really need? If you had to say to somebody very, very rich, indeed, write me a check, which will get this done. How many zeroes on there?
Sue - The human cell atlas project has a budget of about 3 billion dollars for one organism. So at least the same amount as the human cell atlas would be reasonable.
Chris - I hope you are good at writing grants! But some people listening to this Sue may say, "well, this is very laudable that people want to comprehensively map out what all these cells are doing in individual plants and so on, but why should I care?" might be their reaction. So why should a young, up and coming biologist decide to put their career in your hands and do this? Why does this matter?
Sue - Plants are really under-appreciated in our world. The biosphere, as we know today started 3.5 billion years ago by plant ancestors. And agriculture started about 10,000 years ago, which led to the civilisation that we all enjoy today. We will die if plants died. So this planet and everything on it really depends on plants. And now, you know, plants are really becoming the source of bio-renewable energy. And I think in the future plants are going to be a major driver of the economy. I think we're going to have an economy that's based on biology.
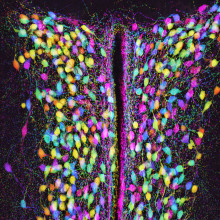
20:39 - Oxytocin: same source, released in two places
Oxytocin: same source, released in two places
Charles J. Frazier, University of Florida
The hormone oxytocin - and its close relative vasopressin - plays, arguably, one of the broadest roles of any signal in the body. Coming from the pituitary gland in the base of the brain it affects how we bond and respond to others, and in the bloodstream it influences salt and water balance, lactation, and uterine contractions. What's interesting is that the same nerve cells make it for all of these different applications, but can release it just where and when it's needed - either centrally for the brain, or peripherally for the rest of the body. Speaking with Chris Smith, from the University of Florida, Charles Frazier explains how he has been finding out how…
Charles - In the simplest sense, what we were trying to find out in this study is how neurons that release a very important peptide called oxytocin regulate the release of that peptide. The relationship between their cellular activity when they're firing action potentials, and when they're likely to be releasing peptide into the brain.
Chris - Because it's a pretty important hormone, oxytocin, isn't it? I mean, it's got effects that manifest both in the brain, but also around the body. And there are various situations when it gets released and they range from people having sex, to breastfeeding, to bonding with a partner.
Charles - Honestly, that's one of the things that was really fascinating to me about oxytocin in general. All of the oxytocin that is active comes from a very small set of cells that lives in just two discrete nuclei in the brain. And that means that the cells that live in those nuclei have to have ways to release the peptide, both into the peripheral circulation, and into the central nervous system. And it turns out that they have overlapping, but distinct mechanisms for regulating those types of release.
Chris - It sounds like a terrific example of efficiency. You use one source of oxytocin, but you've got ways of making it come out in different places at different times. Presumably we don't, or didn't know what those mechanisms were?
Charles - Well, we knew a number of things about what the mechanisms are. And to think about what they are, we have to talk in a little bit more detail about the neurons themselves, and how they're divided into various compartments. So the oxytocin neurons have one structure called an axon, which is the classic kind of signalling structure of a neuron. Yet unlike many neurons, where their axons project to other places in the CNS, a lot of the axons of oxytocinergic neurons project to the posterior pituitary, where they have mechanisms to release oxytocin, right from their axons into the bloodstream. By contrast, there's this other compartment, the dendrites, and the dendrites typically you think of as kind of an input structure, where they receive inputs and integrate them, and decide when the cells should fire an action potential. But in oxytocinergic neurons, not only do the dendrites do that, they also have their own independent ability to release peptide.
Chris - So how do the cells discriminate between, I want to just squirt some oxytocin into the brain, versus I want to deluge the bloodstream with oxytocin. How do they achieve the, the two different effects when they want to do that?
Charles - That's a great question, and very close to the question that originally motivated this study.
Chris - How were you studying it then?
Charles - We were doing in vitro electrophysiology. Making tissue slices through the brain. Taking living tissue slices and putting them under a microscope where we can record from individual genetically identified, oxytocin synthesising neurons. And then we can use a combination of electrical and optical techniques to both stimulate them, and using imaging techniques that let us know when calcium is coming into those structures. And we're using that calcium influx really as a proxy for when they are likely to be releasing oxytocin.
Chris - So have you solved the riddle? Do we now know how it works?
Charles - Well, I'd say that one of the central findings of this study is that there are certain types of signals that act on the dendrites, to modulate the way that they respond to electrical activity in the cell. Signals that can change the amount of calcium influx that shows up in the dendrites, in response to a consistent electrical stimulus in the cell body.
Chris - And what are those external factors that make that difference?
Charles - One type of signal that we studied is called an osmotic stimulus. And in a simple sense, what this is, it's just a stimulus that changes the extracellular salt concentration in the solutions that is around the neurons. And we found that increasing the osmolarity of the extracellular solution decreases the ability of the dendrites to conduct electrical activity from the soma to the likely sites of oxytocin release.
Chris - Okay, so that explains one part of it, but what other factors matter?
Charles - Well, we also found a very common inhibitory transmitter that exists in the brain called GABA, is similarly able to act on the dendrites to modulate the relationship between activity in the soma and calcium influx at the likely sites of oxytocin release. In my opinion, it's plausible that there will be a number of other endogenously available modulators that can act on the dendrites to control this process, that have not yet been discovered.
Chris - Putting all this together. How do you think that this changes our understanding of the physiology of this bit of the body?
Charles - I guess the reason that I think this is really a potentially exciting finding, is because there's a fair amount of interest in targeting central oxytocin receptors to treat a range of conditions that impact mental health. And really doing that is pretty difficult, because oxytocin is not particularly permeant to the blood brain barrier. So if you give it to a human as a medication peripherally, not a lot of it reaches the brain, and the oxytocin that does reach the brain probably doesn't do a fantastic job of mimicking the natural, spatial, or kinetic, or contextual profiles of normal healthy oxytocin signalling. And so the thing that I think is exciting about our findings, is it starts shedding a little light on possible ways to control how the brain releases its own oxytocin, and that might eventually produce a therapeutic strategy that is more effective than bringing in an exogenous bit of oxytocin.
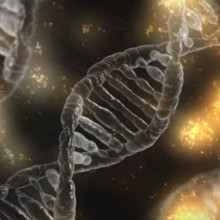
27:02 - Disease-linked genes bog down evolution
Disease-linked genes bog down evolution
David Enard, University of Arizona
Evolution ultimately refines us into the best fit for our environments and the pressures we face living there. So, intuitively, any versions of genes the spell bad news ought to be weeded out over time, leaving behind healthy, unfettered genetic stock. But, an analysis of the rates of evolution of the human genome shows that deleterious - or disease-linked genes - genes don't just bog down their own evolution: they do the same to non-disease-linked genes that sit nearby on the chromosome. From the University of Arizona, and speaking with Chris Smith, David Enard...
David - The way we framed the study was to compare the evolution that happens at genes that have mutations, that in specific environments can cause disease, with the rest of the genome that is not known to be connected to any kind of disease.
Chris - So would a good example of a disease linked gene be, for example, the genes that are linked to breast cancer, the BRCA genes.
David - Yeah, it's actually a good example because BRCA1, well known in breast cancer, is notorious for the precise reason that it carries mutations that make it more likely for women to have the disease at some point in their life. And unfortunately this happens to be mutations that are found pretty commonly.
Chris - Is the question you're therefore asking, if we look at a gene like BRCA, where there are variants, which are linked to deleterious traits like breast cancer, where did those variants come from and how have they affected evolution of genes like BRCA, but also possibly the genes around them.
David - Yeah. And why would we still observe the frequencies of the disease variant that we observe?
Chris - How can you unpick the past in that way though? What's the approach that enables you to sort of see back in evolutionary time in the genome, to see how we arrive at the position we find ourselves in?
David - There are ways to analyze genomes that can tell us how much past adaptation occurred during human evolution. We can then ask if disease genes in particular had different levels of adaptation, compared to the rest of the genome that is not known to be associated in any way to disease.
Chris - In other words, do the disease genes hang around, because they're changing less quickly or holding up evolution of those particular variants, compared with other genes which are unencumbered.
David - This is what turned out from our analysis. What we found was that the presence of harmful mutations can really slow down potential future adaptation. We saw that, basically by seeing that there is a pretty severe deficit of signals of adaptation, of past adaptation, at genes known to be involved in disease.
Chris - So they slow down the evolution of disease linked genes, but they don't encumber other bits of the genome. That carries on evolving, at the normal pace.
David - Not quite as much. So you have to imagine the closer you are to those disease genes, the harder it's going to be for adaptation to happen rapidly. The further away you get on chromosomes from the disease genes, the easier it becomes for adaptation to happen more rapidly.
Chris - This is getting very interesting. So in other words, you've got the gene that is the disease linked gene. And if it has a particular form that causes a disease, it doesn't just slow down its own evolution. It also spills over and slows down the evolution of the bits of the chromosome that are nearby. Why might that be?
David - It happens because mutations that may be advantageous can happen in the same people on the same chromosomes as the disease variant. So you can imagine, if you have an advantageous variant in the future, that may by chance occur in someone with a chromosome that also carries those disease variants nearby on the same chromosome, what happens is that they cancel out the beneficial effect that new advantageous variants might have.
Chris - It's basically like giving concrete shoes to those other genes that are beneficial, and chucking them in a lake isn't it? They're gonna sink because they're dragged down by the deleterious variant that is nearby.
David - Exactly.
Chris - My question would be then, if you've got deleterious variants in the population, why doesn't evolution just take care of them and get rid of them? Surely their frequency will drop to a low level, if they're being so bad, quite quickly, and they'll just get ditched.
David - I mean, it would be great if that was the case. But what happens is that a lot of the disease variants, we know only cause disease if you carry two copies of the disease variants on both of your chromosomes, that come from both of your parents. So it's a matter of bad luck. If both of your parents happen to carry each one copy of the disease variant, and if you have only one copy, the disease doesn't manifest itself. It's only when you inherit two copies of those disease variants that they cause disease. But that means that a lot of those disease variants can stay for quite a long time during evolution in one copy in many people, and being silent in that one copy state.
Comments
Add a comment