This month we’re finding out how genetic advances are shaping the future of healthcare at the Genetics Society autumn meeting. Plus, signposts for bees and an operatic gene of the month. This is the Naked Genetics podcast for December 2017, brought to you in association with The Genetics Society.
In this episode
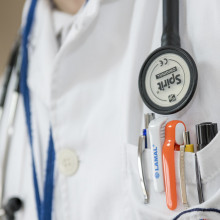
01:04 - Genomics in Healthcare
Genomics in Healthcare
with Michael Simpson, King's College London
Naked Scientist Ginny Smith went along to the Genetics Society Autumn Meeting at the Royal Society in London, held in partnership with the British Society of Genetic Medicine entitled The Human Genome in Healthcare. The meeting revealed how advances in gene sequencing and editing are shaping the future of medical diagnosis and treatment.
First up, she spoke to one of the meeting’s organisers - Professor Michael Simpson from King’s College London and Genomics plc - to find out more about the idea behind the conference and to get a taster of the talks ahead.
Michael - It’s clear with our ability to sequence DNA at scale the human genome is becoming an important part of how we think about healthcare and medicine. Over the next 10 years or so, there's predictions that we’re going to have up to a billion genomes sequenced and most of these are going to be sequenced in the healthcare setting.
There's important challenges in terms of interpreting the variation that we see in various clinical contexts, and I think the coming together of both the Genetics Society membership and the British Society of Genetic Medicine really enables us to think about the fundamentals of genetics in the context of the clinical application of these technologies. We put together a programme which covers a wide range of areas where genetics is going to be important in decision making in medicine.
Ginny - Can you give me some examples of the kinds of talks that you’ve got going on here and the breadth that they're covering?
Michael - This morning we’ve had a session on Rare Disease Genetics – how we can use structural information from proteins to prioritise variants in certain genetic contexts. We’ve got a session this afternoon which thinks about genetics in a slightly different way and trying to treat genetic disorders, and potentially, using the genome as a target for therapeutic approaches with CRISPR based technologies.
And then we’ve got sessions on cancer, surveying of the mutations arising cancer can be useful for both predicting prognosis and response to therapies, and then the potential for genetics to be used in the context of common complex disease, and also pharmacogenetics - how individuals, based on their genetics will vary in their response to certain drugs.
And then we’ve also got the final session tomorrow afternoon, which looks at some of the complex ethical issues of using this kind of data and also, the educational side of this in terms of bringing the public, raising their awareness of what genetics is, the promise that it holds, but also the challenges that we’re currently facing using this type of data effectively.
Ginny - What do you think is the most exciting development that we’re going to see in healthcare in the next say, 10 years that’s based on kind of genetic research that’s going on at the moment? Is there an area that you're particularly looking forward to seeing where it goes?
Michael - So, I think there's two parts. There's the day-to-day use of this kind of technology in the clinic. And so it’s used, particularly in cancer, in trying to identify which individuals are going to respond to certain therapeutics.
But also, that we can actually use this information once we have it at scale and a greater understanding of the relationship between genetic variation in human disease, and to prioritise targets for the new therapeutic development and help decrease the risk of some of these targets in drug development programmes.

Drowning in data
with Kaitlin Samocha, Wellcome Trust Sanger Institute
Before doctors can use genetic data to diagnose and treat disease, they need to know exactly what the myriad different variations in the genome mean for sickness and health. And as the amount of genetic data expands exponentially, that problem is only getting bigger. Kaitlin Samocha, from the Wellcome Trust Sanger Institute, is trying to get to grips with the data deluge and sift the signal from the noise - as she explained to Ginny Smith...
Kaitlin - One of the big questions within genetics is being able to understand any genetic changes that we see in individuals, and what you need to be able to do to understand the change in one individual is to look at many, many individuals. And the reason this is important is because all of us have a lot of changes in our genome. And so, we really need to be able to filter out the changes that are important from the changes that are there because they're there, because you're human, because you have them.
There is a subset of changes within each individual that might be contributing to differences and their height or their weight. And we want to be able to determine which changes are contributing to the height difference and which changes aren't contributing. And so, in order to be able to understand that, it’s best to look at many people.
The work I've been doing is looking at many people to come up with ways to separate out the signal from the noise, the interesting changes from the not-so-interesting changes, if you want to summarise that way.
Ginny: And how are you applying this to healthcare?
Kaitlin - So, the application to healthcare is that we know genetic changes can influence your risk for disease or a particular disease that you have. If you look for example at children with developmental disorders such as severe intellectual disability, we know that there are genetic contributions to that.
What we want to be able to do is when we look at one of these children and we have all of their genetic changes, we want to be able to find the subset of changes that appear to be contributing to their developmental disorder as opposed to contributing for example to their height.
Ginny - So there’ll be some differences in the genome of someone with a disease that are causing the disease perhaps, but then there’ll be other differences that are just at random. Is that the idea?
Kaitlin - Yes, that’s the exactly the idea. There are plenty of changes within every individual that don’t have a major impact at all. They seem to be relatively neutral in terms of the way that the individual acts or operates overall.
Ginny - If you’ve got, say, a sample with a disease and you find a change in their DNA, how do you then work out if that is the change that’s causing a problem or just something that’s come up at random?
Kaitlin - That’s an excellent question. So you have an individual and you have all their variation. The best way to understand any changes you’ve seen in an individual is to look at a population. So, you can check, for example, if anyone else with a very similar disease has that exact same change. And that is one of the ways that you can say, “Well, if two people with the exact same disease have the exact same change, that seems interesting.”
Another way to do it is to look at relatively healthy people – the general population – and to say, “Let’s find areas that no one has a change in” because if you have this particular gene for example that makes a specific protein, if you look at most of the population, they don’t have any changes in this gene at all, but your patient has change, that indicates to you that most people seem to be intolerant of any change here where this change might be more likely to be leading to their disorder.
Ginny - But am I right in thinking that most diseases, it’s not as simple as a single gene that will have a change? It’ll be a combination of genes.
Kaitlin - Correct. That’s what makes it even harder – every individual for many things will have a variety of changes, multiple changes that are all contributing. And so, what we’re trying to do is help highlight the areas or the specific changes that appear to be more likely to be important. And so, you could do this across the board and if someone has five changes that are all never seen in a healthy person, those five changes might be working together in order to lead to the disorder. One of the main challenges today is not only to highlight changes that look interesting but to figure out how they're working together to lead to some of these different disease states.
Ginny - Now this sounds to me like it might be as much of a data issue as it is a biology issue. How do you handle the quantity of information you must have to deal with on a daily basis?
Kaitlin - We have some very nice computers! And actually, increasingly, people are moving to cloud-based systems. So instead of storing for example, all living individuals’ changes in some text file on your computer, we store it across many computers that you can access from many different places in the world and this allows researchers not only at the Sanger Institute where I'm based but researchers in Boston or in Germany, or variety of places in the world to all contribute understanding the different changes in how they work together.
Yes, this is becoming a computational issue and increasingly these days, we’re shifting away from looking at an individual person on their own and trying to solve one thing at a time. We’re looking at large scale data altogether to understand. So yes, programming and computer skills are increasingly important biology these days.
Ginny - And what kind of numbers are we talking about in these population studies?
Kaitlin - So a data set I've worked with a lot has 61,000 relatively healthy people. We’ve now released a data set that’s roughly 140,000 relatively healthy people. The UK Biobank which is a big study based of course in the UK has 500,000 people. So, as we continue to sequence or look at different genetic changes in individuals, it will scale up to hundreds of thousands and millions of people. And that’s what we need if we want to put single individuals in the context of millions.
Ginny - And how do you feel your work is going to apply to the future of healthcare in general?
Kaitlin - Many people have predicted that in the somewhat near future, every individual will have their genetic variation interrogated. So you might be walking around with knowledge about all of your genetic changes. The hope is to be able to educate clinicians so that they can help use this information in order to prioritise what you specifically have.
So we already know for example that there are some genetic changes associated with how well you process particular drugs. That’s incredibly important for your GP to know before they give you any particular drug. In the future, the hope is that the work that I've done will give the clinicians a way to prioritise what might be involved in your particular disease that you're seeing your physician or your clinician about generally. That’s always the hope!

Genomes - thousands of them
with Richard Scott, Genomics England
One of the biggest research programmes in genomic medicine in the UK right now is the 100,000 Genomes project, run by Genomics England. Ginny Smith spoke to Richard Scott from Genomics England to find out more about the project and how it could change medicine in the future, particularly for rare diseases.
Richard - So the project aims to recruit patients from the NHS. A total of 100,000 genomes of people who have either cancer or rare disease to both help their own clinical care in the NHS but also, allow research and to foster a genomics industry in the UK.
Ginny - Why is it so important to know more about the genetics of cancer and these other diseases?
Richard - So both cancer and rare disease are genetic diseases in different ways. Cancers are genetic in that they form because of mutations in the cancer cells that are different from the cells that you're born with. Rare diseases can quite often be caused by very rare genetic alterations in the genes, in the DNA that you're born with. If you understand the cause either in cancer, what's driving it, or in the rare disease, what's caused it, it helps you look after the person better.
Ginny - There must be specific challenges to working with rare diseases in that you have fewer people to work with. How does that affect your project?
Richard - So rare disease is strange. Each of the individual diseases is rare. So by definition, there's 1 in 2,000 or fewer people are affected with it. But collectively, they're very common so there are thousands of different rare diseases. So we think about 1 in 17 of the population actually have a rare disease.
The real challenge is, we’re actually in medicine, quite poor at recognising many of these diseases. So taking a broad diagnostic approach as you can with genome sequencing is really helpful in many of these situations because it helps you to find the cause where using the most traditional approaches is really challenging. It’s like finding a needle in a haystack sometimes and these newer technologies really help with that.
Ginny - I guess 1 in 2,000 is rare for a specific GP to have come across that disease before. But actually, in terms of the world population, there are quite a lot of people living with each of the diseases.
Richard - That’s right for those that are as common as 1 in 2,000 and in the rare disease, well that feels common. There are a lot of people with those, but we know that there are many diseases which are really vanishingly rare. We’re very lucky in this country that we’ve got a project of such scale.
100,000 genomes is a lot but even with that, it’s too small for us to know that we’ll find the causes of some of these really vanishingly rare conditions where perhaps will only recruit one person with the condition to the project. It is very hard to recognise patterns when you're only looking in one person in one family.
Ginny - So if you were say, a person with one of these really rare diseases, is there still going to be a benefit to you from being part of the project?
Richard - Absolutely. So, our primary aim in terms of the immediate benefits to people with rare diseases joining the project that we might find the diagnosis. We know that we can't be sure whether or not we will, but that’s the prime initial aim. It also means that potentially in the future, if a cause is found or something else recognised in the genome that is useful clinically, there's a potential for the people to be recontacted and offered the possibility of recruitment to other research to do with their condition that might be helpful to them themselves, or to other people with their condition in the future.
Ginny - And as well as diagnoses, will there be effects that might change their treatment if they’ve been part of the project both for the rare diseases and for the cancer patients?
Richard - Absolutely. In cancer particularly, the focus is on changing treatments whether that’s avoiding treatments that you can see from the signature in the cancer wouldn’t be effective but might just have unnecessary side-effects or whether it’s finding something quite targeted that will be more effective.
In rare disease, there's changes in treatments although that’s sadly at the moment, a relatively small number of rare disease is not possible. But it’s often possible to change management. So for example, to know in someone, if you know what exact disease they have, whether you should look out for particular medical complications more carefully than in a general population - perhaps screen them for high blood pressure at a younger age then the general population would need. That sort of thing can be really helpful and actually change outcomes quite a lot.
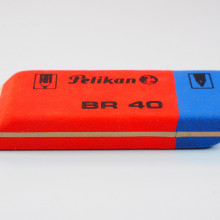
16:03 - Genome editing: Cut and paste
Genome editing: Cut and paste
with Andrew Wood, University of Edinburgh
Every year the Society gives out a number of prizes to leading geneticists, who win the opportunity to give a lecture about their work. Andrew Wood, from the University of Edinburgh, is the winner of this year’s Balfour lecture, given to an outstanding young investigator. Here he is talking to Ginny Smith about his prize-winning work...
Andrew - CRISPR-Cas9 is a new tool. It was developed for use in human cells in 2012. However, it replaced older, clunkier versions of technology that aim to do essentially the same thing. and part of what I was talking about today was my experience with some of those older technologies and comparisons between those and modern-day methods.
Ginny - So what kinds of sequences might you be wanting to add to or take out of the genome and why?
Andrew - Well for example, let’s imagine that a clinician has identified a human disease and a gene variant has been found in individuals who have that disease. In order to show that that gene variant is causal for the disease, one of the approaches that researchers take is to take cells and engineer that particular variant into a cell that didn’t have it previously and to see whether or not that cell behaves differently in a way that might link that variant to the disease identified in the human.
Ginny - So you can actually kind of create models of these diseases in animals?
Andrew - In animals, in human cells, essentially, any living organism now. That’s one of the great things about genome editing, that it is portable between just about any living system that you can imagine.
Ginny - And I guess that then means that you can test drugs and other therapies, and see if you can cure the disease that you’ve created?
Andrew - Absolutely. It means that you can create for example, cellular models that have these variants and then screen compounds or drugs and see how cells that have that variant behave and how that differs from cells that don’t have that variant. That would be one approach that a lot of people are taking.
Ginny - Is there anything in particular that you’ve come across so far in this meeting or that’s coming up tomorrow that you think is really exciting and you think might be the kind of the next step in genetics and healthcare?
Andrew - The general theme of mining large quantities of DNA sequence data in order to find out what distinguishes populations of individuals with respect to their susceptibility to diseases I think is really exciting.
Ginny - So looking at the kind of preventative or predicting disease before it even happens rather than curing it after it’s happened?
Andrew - Yeah, exactly that. Also, perhaps kind of retrospectively looking at the way that different groups of patients have responded to a particular drug and seeing whether or not those which responded in a positive manner have particular variants that are not present in the group that didn’t respond well to the drug for example.
Ginny - So this is coming to us to a kind of idea of personalised healthcare that we’ll all be screened and whatever treatments we’re given will be tailored for us.
Andrew - Absolutely, yeah. That’s a big buzz word in medicine at the moment.
Ginny - Do you think it’s likely that that’s going to be happening soon or is it still a long, long way away in terms of being financially viable?
Andrew - Well, I mean there are a few sort of poster child cases that get kind of trotted out as a good test case for this kind of approach. It is inherently a very expensive business. First of all gathering the genetic information is very expensive. Any kind of drug development process is also incredibly expensive. So yes, the idea of this leading to therapies quickly that can be applied to lots of people at the moment still seems quite a long way away.
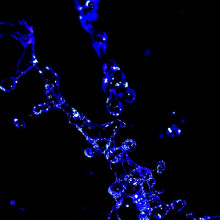
20:01 - Fixing our genes
Fixing our genes
with Jakub Tolar, University of Minnesota
As you’ve probably guessed from the number of times we’ve covered it on previous podcasts, there’s a huge amount of excitement about the potential for CRISPR to treat human diseases. But what are the most promising applications? To find out, Ginny Smith caught up with Jakub Tolar, from the University of Minnesota...
Jakub - There are number of early adopters of this technology and one of the leading technologies is called gene editing. It basically means that you have a typo in the three billion letters of human genome and you just take that single letter that is incorrect and you exchange it for the right one.
Ginny - How do you go about doing that?
Jakub - It is absolutely fascinating because we have used what I would call “augmented reality” for this because we have generated molecules that don’t exist in nature and put together two functions that do exist in nature: One that binds DNA - that binds to a specific site in the DNA - and the other one that cuts DNA. So we put them together and these hybrid molecules, they don’t exist in nature, have been used in service of the gene editing, of the rewriting of the incorrect information that is in the blueprint of the genome.
Ginny - How do you get these brand new molecules into a living human?
Jakub - You are asking exactly the most important bottleneck of the whole technology, because the cell is very smart. It defends itself from any foreign DNA or any foreign particle such the ones that we would be using for gene editing. So to accomplish this, we are trying to be also smart and learn from nature. So we for example, modify the nucleic acids that we use for this or we encapsulate some of the proteins that we want to deliver so that it can cross the cellular membrane as well as the nuclear membrane, and gets to the heart of the nucleus where the DNA lives.
Ginny - You're effectively hiding it so that the cell can't tell that it’s something that it shouldn’t be letting in?
Jakub - Yeah, that’s a good term. We are effectively hiding it from the defence mechanisms that exists in each cell.
Ginny - This sounds really kind of sci-fi futuristic. Is this something that’s actually currently in use?
Jakub - Frist of all, there's nothing sci-fi about this. There are no miracles. I always cringe a little bit when I see “this is a medical miracle”. It almost never is. It’s a sequence. It’s a readout of a year or a decade long work of many, many people. So I think this is a predictable, incremental, very exciting pathway we are taking now.
Ginny - What kind of diseases is this currently being used for?
Jakub - The majority of the disorders that this would be helpful for are monogenic disorder. What that means is that a single gene is faulty in the whole genome of that individual that’s born with that disease. It would not be used at this time for what we call polygenic disorders which is many, many genes are affected by the disorder.
The examples of the diseases that this would be helpful for would be, for example, bone marrow failures. This is a state when kids are born with insufficient number of stem cells that are blood forming in the bone marrow and will eventually use them up and have no more to provide for healthy platelets and white cells and red blood cells. It can equally be used for liver disorders that are hampering the ability of the liver to detoxify or make new proteins that are necessary for healthy living. And as I showed in my comments, it can be used for skin disorders such as epidermolysis bullosa. It’s a very, very hard disorder even for some people to look at, but it’s a very equally important – at least for me – it’s a challenge for medicine and science and medicine to approach. And the hallmark of the disease is loss of skin. These are similar to a chemical or thermal burn and only this time, the burn is genetic.
Ginny - And this disease can be treated using these new molecules that you’ve created?
Jakub - That’s what we are working on. That is where we are headed.
Ginny - So how would the process actually work if you were to go in for gene editing treatment? What would it be like as a patient?
Jakub - So I think that the incremental safety levels needed that needs to be cleared I think are that these cells, skin cells, and perhaps other cells that make these all-important skin molecules (they don’t have to live in skin) called mesenchymal stromal cells will be corrected outside of the body of that individual and then her or his own cells will be given back to them.
Ginny - Okay, so the novel molecules wouldn’t actually be going into the body. That will be done outside.
Jakub - I would prefer that because I think it’s a whole new box of Pandora to go into the whole organism with a genetic medication.
Ginny - What could be the risks of this kind of gene editing technology? Is there a danger that it could edit in the wrong place for example and cause problems?
Jakub - You are very smart, yes! That is the major concern of ours and everybody else working in the field that inadvertently, the same mechanism that can lead to clean correction where we desire it can lead to undesired “correction mistakes” introduced somewhere else in the genome that would have clinical consequences.
Ginny - And what kind of effects could that have in theory?
Jakub - The most feared one is cancer. It has happened in the past with other forms of gene therapy. So people are especially sensitive to this and rightly so. Another one would be dysregulation of the human immune system. I can see that this can lead to autoimmunity problems as well.
Ginny - Do you think that this kind of technique will be developed and will be able to be used for more complex disorders that involve multiple genes or will they always too complicated?
Jakub - No. I am almost certain that this is headed to the direction that as we perfect it on the level of a single gene, this will advance to the polygenic disorders as well. One of the great advantages of this approach is that it’s modular. It can be like Lego. It can be put together from different pieces and you can combine different corrective molecules on the same molecule in the same cell.
Ginny - Now the million-dollar question: How long until these kind of technologies are going to be in widespread use?
Jakub - Million-dollar question but no answer because I think that there's a reason for why the future is unpredictable. We just don’t know. But if I take it from other parts of technology such as transistors - from a transistor in Bell’s labs to the transistor radio from Texas instruments, it’s about 10 years...
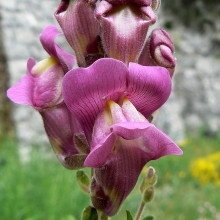
Signposts and snapdragons
with Enrico Coen, John Innes Centre, Norwich
Professor Enrico Coen from the John Innes Centre in Norwich has published a new paper in the journal Science, revealing a fascinating genetic mechanism underpinning the evolution of the brightly coloured ‘signposts’ that bees use to home in on the juicy nectar in the centre of snapdragon flowers. Kat Arney caught up with him for a chat about his latest floral findings.
Enrico - In the Pyrenees, there are these two species of snapdragons that have different ways of signposting to bees where the bees can enter the flower. So there's a sort of like a red or magenta flower with a yellow highlight and that’s one species, and then there's another species with yellow flowers and a magenta highlight.
We were trying to understand how these differences arose. I mean, why does one species, one type of signposting species have another type of signpost? And to do this, we started to analyse some of the genes involved that control these different traits. The amazing was that we found out that one of the genes, the genes that controls the yellow highlight arose through a sort of very strange mirror image duplication. So it sort of created a mirror copy of itself.
And through this mirror copy it generated a specific type of molecule called a small RNA, and that molecule is what's involved in restricting the yellow pigmentation. So that was a complete surprise.
People had found these types of mirror image duplications in the laboratory and then we know they're also part of the genome. But to discover that they were responsible for this variation that you see out there in nature, that was fantastic surprise really and that’s what the paper is about, about that discovery.
Kat - When I think back through my history of genetics, I do remember that the first discovery that small bits of RNA could turn genes off was made in petunias. Researchers were trying to make the purple colour in petunias and then they discovered that they accidentally switched the purple gene off. So is that sort of a similar process and is that process widely used in plants?
Enrico - It is a similar process. The petunia story you're talking about is when people were putting genes into petunias artificially. So they were inserting these genes into these plants, and discovered this strange phenomenon. And this is exactly the phenomenon that we’ve discovered except that it’s not through people changing artificially the genomes. It’s happening in nature itself. So that’s the striking thing that actually nature was there way before us in terms of these types of rearrangements and mirror images.
Kat - What's the next step for this now that you’ve discovered this? What are you going to do with the information now you know there is this kind of mirror image thing that switches the colour off?
Enrico - So what we’d really like to know now is how this whole system originated. What we’re seeing today is different signposts. We’d really like understand how did it arise: how did it, how is it that the evolution alone looks at one signpost, one species, another signpost in the other species?
And that’s looking, digging deeper into the genome, looking at how these originated, how these mirror image duplications arose, really looking quite broadly across the different species across their genomes, trying to do experiments to figure out how this remarkable difference arose. It’s a bit of a conundrum. How is it that you end up with two equally good solutions starting from one beginning?

Gene of the month - Pavarotti
Named after the famously portly opera singer, the Pavarotti gene was first identified in Drosophila fruit flies in 1998. Fly embryos with a fault in their Pavarotti gene have abnormally large cells in the nervous system, because they fail to divide properly and just keep on growing. Eventually, the embryos die because they can’t form a proper nervous system.
Further research revealed that Pavarotti encodes a type of protein that acts as a tiny molecular motor, known as a kinesin. These motors crawl along the biological scaffolding inside cells and help to change a cell’s shape and structure - vital steps in the process of cell division as a cell contracts in the middle and splits into two.
In mammals, Pavarotti is known by the less dramatic name mitotic kinesin-like protein-1, or MKLP-1. But rather than being responsible for knocking out a passable version of Nessun Dorma, it’s also involved in building healthy nerve cells.
Comments
Add a comment